If the earth were completely covered with water, the sea level would spread out along an irregularly shaped surface. This surface deviates by up to 100 m from a flattened sphere and is scientifically known as the geoid. If these deviations are greatly exaggerated, the earth looks more like a potato. This is where the colloquial name “Potsdam gravity potato” comes from, which honors the decades of work on determining the geoid and the earth's gravity field on the Telegrafenberg in Potsdam.
Dr. Bernhard Steinberger, GFZ
One of the features of the geoid that immediately catches the eye is a pronounced “dent” in the Indian Ocean. This is partly due to the fact that the geoid is usually represented relative to the reference figure of an ellipsoid that fits best overall. It would make geodynamic sense to represent it relative to the equilibrium figure: the earth is actually flattened by several tens of meters more than would be the case in equilibrium. Thus, using this equilibrium figure, the dent is slightly less prominent but still visible and is now part of a geoid “trough” that extends from pole to pole (Fig. 1).
The geoid's deviation from the equilibrium figure is caused by additional or missing masses of the solid earth: Additional mass - material with a higher density or higher topography - causes additional gravity. This attracts the seawater more strongly and the geoid develops a bulge. In the same way, missing mass causes a dent.
At what depth of the earth is the mass missing?
So far, so simple, but if you want to find out exactly at what depth the mass is missing, it becomes more complicated: A hot, less dense upflow in the Earth's mantle (missing mass) also causes the surface to be pushed upwards (so-called dynamic topography; additional mass). These two effects partially compensate each other. Which effect is dominant depends on the depth of the mass, the spatial expression of the signal and the viscosity in the Earth's mantle. We only know very imprecisely about the latter, since even the deepest boreholes are limited to the Earth's crust, which is between 5 and 70 km thick. However, it is widely assumed that the lower mantle is on average about 30 to 100 times more viscous than the upper mantle. With such a viscosity distribution, the regional dent may be caused by a lack of mass in the upper part of the Earth's mantle (depth range of about 300 to 1000 km). The large-scale trough on which it rests, however, could be caused by a comparatively higher density in the lower half of the Earth's mantle (down to about 2900 km depth).
Currents in the Earth's mantle as the cause of the dent?
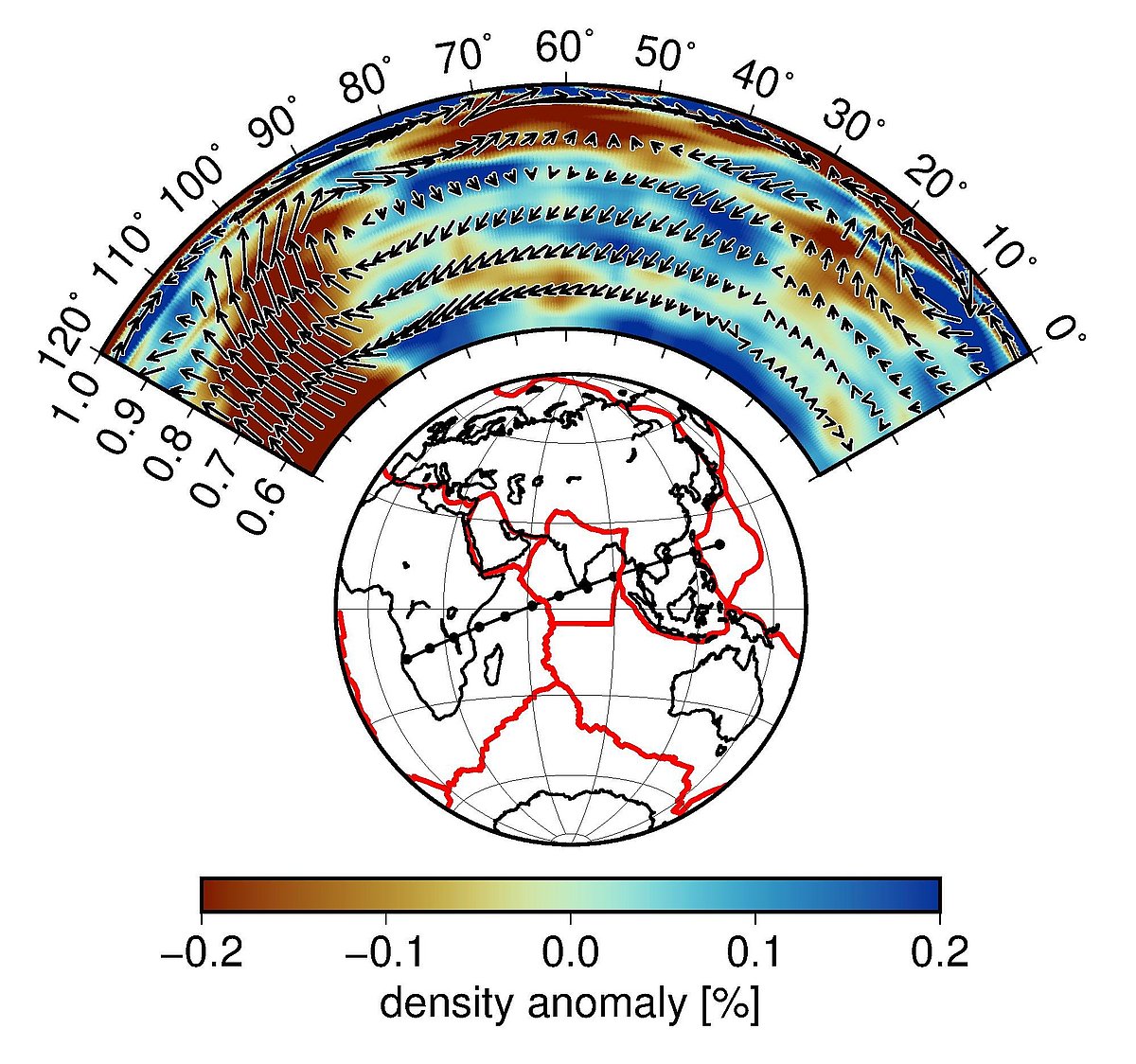
© Bernhard Steinberger
If seismic velocities are converted into temperature and density anomalies, this is exactly the distribution that results: The large-scale mantle structure with two antipodal zones of reduced shear wave velocities under Africa and the Pacific, which presumably correspond to hot upflows, has long been known. In between there is then a cooler downflow, where the geoid trough is located. Newer tomography models now also show reduced shear wave velocities in the upper mantle in the area of the dimple.
But what exactly could be the cause of this presumably hot material? It is possible that material from a hot upcurrent under East Africa, which causes volcanism there and pushes the Earth's crust upwards, is being deflected eastwards in the large-scale mantle wind towards the convergence zone of the Eurasian and Indo-Australian plates (Fig. 2).
However, a new publication (Pal and Ghosh, 2023) has now also shown that there may not be any need for a missing mass in this area: In the uppermost mantle down to about 300 km depth, the relationship between masses and geoid is reversed again: A missing mass here creates a bulge in the topography as well as in the geoid.
It could therefore be that the dent is "only" part of the large-scale trough, but is separated from the rest of the trough by zones of comparatively less negative geoid north and south of it, caused by negative mass anomalies in the uppermost 300 km. The thickened crust beneath the Tibetan Plateau would be the most obvious main cause of the missing mass north of it, while south of it the cause could be a local upflow in the mantle, a so-called mantle plume, whose hot material is distributed under the lithosphere.
Surprisingly, this would mean that there actually doesn't have to be anything underneath the dent: the large-scale subsidence is already caused by the fact that there must be a downward flow between the two antipodal upflows, and the supposed local dent only looks like that because there are bulges to the north and south of it.
Research into the Earth's gravity field on Potsdam's Telegrafenberg
Today, work is still being carried out on the Telegrafenberg in Potsdam to measure the Earth's gravity field. Satellite missions such as GRACE and GRACE-FO now observe temporal changes in the gravity field that are due to mass redistributions in the oceans and the continental hydrosphere, making it possible, for example, to observe groundwater reservoirs from space. At the same time, however, researchers at the GFZ are also developing geodynamic models of mantle convection, which are used to model the geoid in other regions, such as the Ross Sea GeoidThe geoid is an irregularly shaped reference surface in the Earth's gravitational field, at which all points experience the same gravitational potential (equipotential surface). It is therefore a theoretical reference surface that is represented to a... Low, the deepest geoid depression in the world compared to the equilibrium figure (Steinberger et al., 2023). Other applications include, for example, modeling paleogeography (ocean cover, land bridges, ocean currents, etc.; Straume et al., 2024) or the breakup of continents (Brune et al., 2023).
References
- Sobolev, S. V., Steinberger, B. (2012): Geodynamische Modellierung: Zusammenhänge zwischen Struktur der tiefen Erde, Vulkanismus und Umweltkatastrophen. - System Erde, 2, 2, 56-61. https://doi.org/10.2312/GFZ.syserde.02.02.9
- Steinberger, B., Gassmöller, R., Trumbull, R., Sobolev, S. V., Weber, M. (2014): Manteldynamik und das Aufbrechen von Gondwana. - System Erde, 4, 2, 14-19. https://doi.org/10.2312/GFZ.syserde.04.02.2
- Brune, S., Kolawole, F., Olive, J.-A., Stamps, D. S., Buck, W. R., Buiter, S., Furman, T., Shillington, D. J. (2023): Geodynamics of continental rift initiation and evolution. - Nature Reviews Earth & Environment, 4, 235-253. https://doi.org/10.1038/s43017-023-00391-3
- Pal, D., Ghosh, A. (2023): How the Indian Ocean geoid low was formed. - Geophysical Research Letters, 50, e2022GL102694. https://doi.org/10.1029/2022GL102694
- Steinberger, B., Rathnayake, S., Kendall, E. (2021): The Indian Ocean Geoid Low at a plume-slab overpass. - Tectonophysics, 229037. https://doi.org/10.1016/j.tecto.2021.229037
- Steinberger, B., Grasnick, M.-L., Ludwig, R. (2023): Exploring the Origin of Geoid Low and Topography High in West Antarctica: Insights from Density Anomalies and Mantle Convection Models. - Tektonika, 1(2), https://doi.org/10.55575/tektonika2023.1.2.35
- Straume, E., Steinberger, B., Becker, T.W., Faccenna, C. (2024): The impact of mantle convection and dynamic topography on the Cenozoic paleogeography of Central Eurasia and the West Siberian Seaway. - Earth and Planetary Science Letters, 630, 118615, https://doi.org/10.1016/j.epsl.2024.118615